Flexible electronics take giant leap with new crystal production method
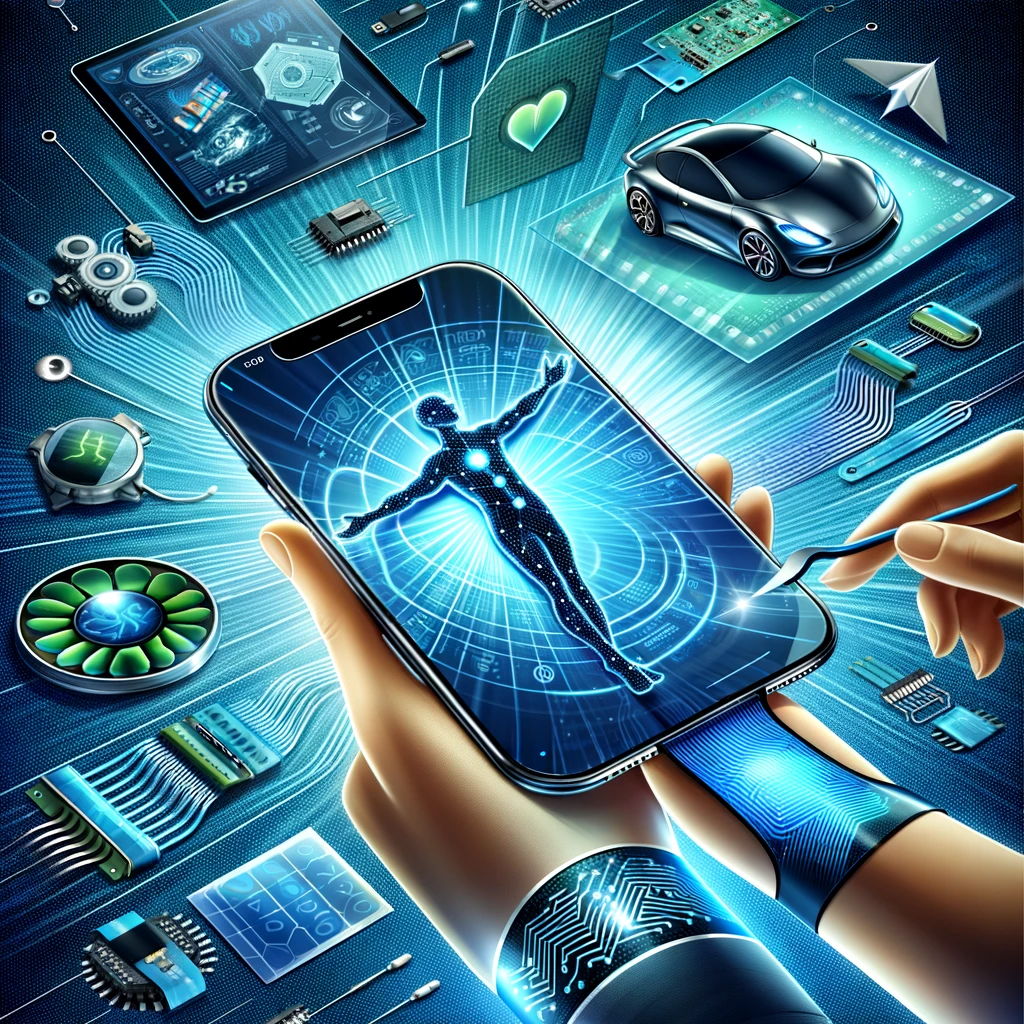
Researchers at the University of California, Irvine (UCI) have developed a novel method for creating ultra-thin crystals of bismuth, a metal with unique properties that could revolutionize how cheap flexible electronics are manufactured.
Bismuth, a heavy metal with a shimmering rainbow-like quality, has captivated scientists for over a century because of its low melting point and unique electronic properties.
"Our new method not only allows us to create incredibly thin bismuth crystals but also reveals previously hidden electronic behaviours on the surface of the metal," explained Dr Javier Sanchez-Yamagishi, assistant professor of physics & astronomy at UC Irvine and co-author of the study.
The team developed bismuth sheets just a few nanometers thick - a mere fraction of the width of a human hair. This remarkable feat unlocks the potential for a new generation of flexible electronics.
Theorists have predicted that bismuth possesses special electronic states that allow it to exhibit magnetism when influenced by an electric current - a property crucial for the development of quantum electronic devices, which rely on the manipulation of the magnetic spin of electrons.
The team was able to observe a hidden behaviour called quantum oscillations originating from the crystal surfaces. Quantum oscillations were initially discovered in bismuth during the 1930s, but they have not been observed in nanometer-thin bismuth crystals until now.
According to Laisi Chen, a Ph.D. candidate in physics & astronomy at UC Irvine and one of the lead authors of the paper, quantum oscillations originate from the crystal surfaces. These oscillations arise from the movement of electrons within a magnetic field, it can exhibit effects that are important for the performance of electronics.
Interestingly, the production method draws inspiration from a common kitchen appliance. Amy Wu, a Ph.D. candidate in physics in Sanchez-Yamagishi’s lab, compares the process to using a tortilla press. To make the ultra-thin sheets of bismuth, the researchers had to squish bismuth between two hot plates with atomically flat surfaces.
"We then made a kind of quesadilla or panini where the bismuth is the cheesy filling and the tortillas are the atomically flat surfaces," Wu explained.
After spending over a year making these thin crystals, the researchers were surprised by the results. "We had no idea whether its electrical properties would be something extraordinary. When we cooled down the device in our lab, we were amazed to observe quantum oscillations, which have not been previously seen in thin bismuth films," Sanchez-Yamagishi stated.
The researchers believe this method can be generalized to other materials with low melting points, such as tin, selenium, and tellurium, potentially revolutionizing the way we manufacture electronic circuits in the future.
"It could be interesting to explore for future flexible electronic circuits," Sanchez-Yamagishi concluded.
This remarkable feat opens a new frontier in electronics, allowing for the creation of flexible and lightweight devices with unprecedented capabilities.
What's next?
The research team now plans to explore further applications of this compression and injection molding technique in developing the next generation of computer chips for smartphones and tablets.
The team is currently working on refining our techniques to gain even greater control over the shape and thickness of these bismuth crystals, which they believe will ultimately streamline the device fabrication process and pave the way for mass production.
The study titled "Exceptional electronic transport and quantum oscillations in thin bismuth crystals grown inside van der Waals materials" was published in Nature Materials.
The team behind this discovery included collaborators from UC Irvine, Los Alamos National Laboratory and the National Institute for Materials Science in Japan. The research was primarily funded by the Air Force Office of Scientific Research, with partial support coming from the UC Irvine Center for Complex and Active Materials Seed Program, a Materials Research Science and Engineering Center under the National Science Foundation.
- READ MORE ON:
- Bismuth crystal
- Flexible electronics
- Quantum oscillations